ewoodrick
Well-Known Member
There is a very good match between the general tests of for example Panasonic cells with NCA chemistry and the Panasonic cells Tesla use.
Actual Tesla model 3 cells. Only three test points but if you take these and overlay on the multi point tests you get a descent match.
Actual Tesla model S cells calendar aged:
These arecells taken out of almost brand new Teslas.
Here’s the sum of the research data set as the blue line, for my MSP’s average cell temp and the amber line is the monthly Nominal Full Pack. So far a very good match.
(I made the same for my M3P 21 during 2.5 years and it was a very good match as well.
I find it slightly interresting that people driving EV’s and that have at least slight interrest in EV technology which to a very high level is built on research,
does’nt accept the research about lithium batteries![]()
That wasn't so hard was it.
So, to summarize, we're are only talking about 3, maybe 4% differential in initial decline. And none of the testing seems to be long enough to measure the much more important period of stability after the initial degradation.
Aren't we talking about similar differences when we talk about the wheel covers on and off with a Model 3?
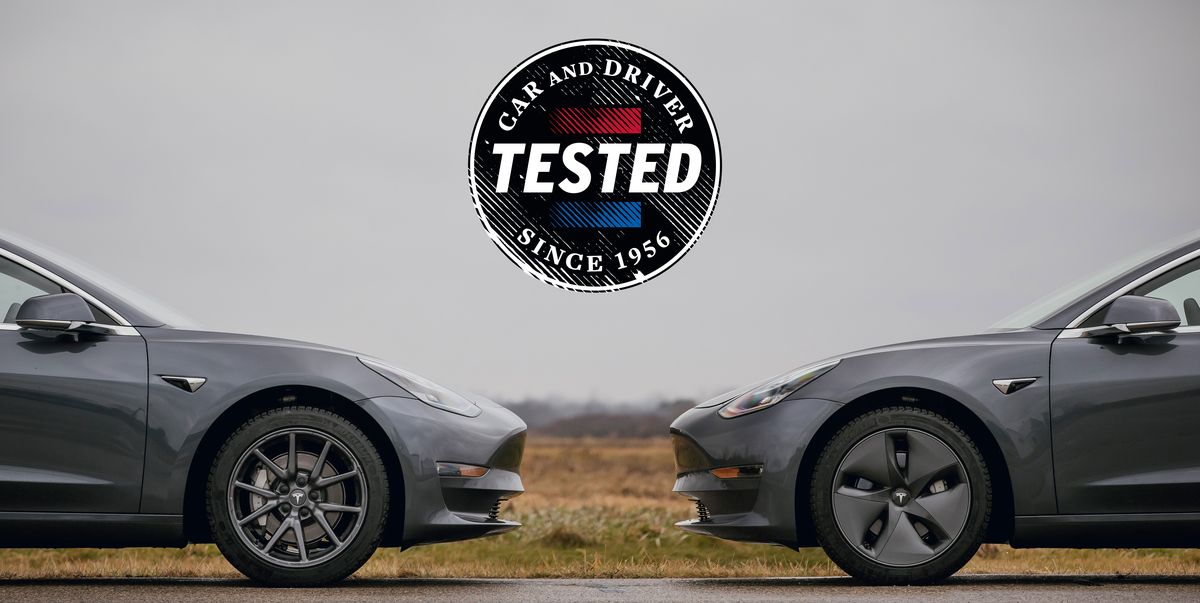
Our Testing Shows Tesla Model 3 Aero Wheel Covers Really Do Improve Efficiency
In our testing, they proved their worth by yielding up to 10 additional miles of range.
Last edited: